Projects
Below is a description of the broad themes of ongoing studies in the lab. New projects are being developed in collaboration with and by trainees and staff in the lab, as we make new discoveries.
Immune Responses to Borrelia burgdorferi Infection
Infections with Borrelia burgdorferi cause Lyme disease in humans. B. burgdorferi is transmitted through the bite of an infected tick. Ticks are first infected when they bite infected small rodents, deer mice, house mice, and other species, such as voles and small birds. These species are considered “natural reservoir hosts” for B. burgdorferi because this is where B. burgdorferi establishes persistent infection from which it is transmitted into biting ticks.
It is important to note that, though these animals are persistently infected, they do not develop disease and thus continue to act as an effective reservoir for the bacteria to spread. We aim to understand how the immune response is altered or suppressed to allow persistent infection to occur.
Understanding these processes could lead to the development of therapies that could bolster the immune response to B. burgdorferi in humans, clearing the infection without the need for, or in combination with, antibiotics. It may also help us understand why some humans continue to have symptoms of infection despite antibiotic treatment (the so-called post-treatment Lyme disease syndrome, PTLDS), which is an outcome analogous to “long COVID”.
We use mouse models for most of our studies. Our studies, and those of other labs, have provided evidence of Borrelia-specific adaptive CD4 T cell and B cell immune response induction and the development of robust antibody responses. However, both CD4 T cell and B cell responses show abnormalities that may explain why effective immunity to this pathogen is not induced (Tracy KE and Baumgarth N, 2017; Hammond et al. 2023). Projects in the lab are focused on defining the molecular mechanisms and functional outcomes of the abnormal T and B cell immunity and the use of proof-of-concept treatments to convert ineffective into effective adoptive immune response induction.
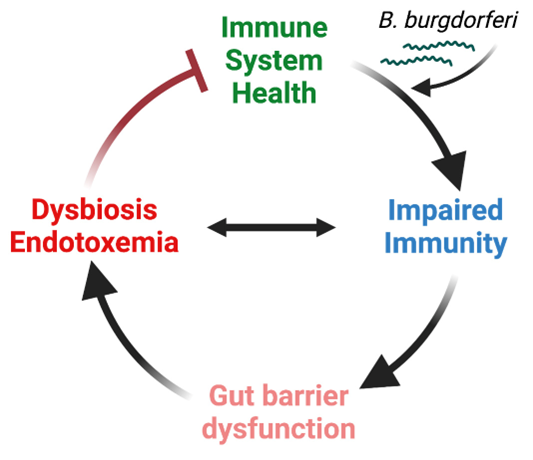
Recently, we discovered that B. burgdorferi alters the gut microbiome of infected mice, suggesting profound changes to the interaction of the host within its microbiome and the host metabolism—likely an outcome of the continuous growth of B. burgdorferi in the host, as these bacteria rely on the host for much of their nutritional needs (Sachan, in prep 2024). These are exciting findings as they offer potential explanations for some of the symptoms human patients are experiencing. We are seeking to understand the mechanisms causing these changes to the gut and its microbiome and determine whether the changes to the microbiome may benefit B. burgdorferi.
Fig. 1 Working hypothesis B. burgdorferi infection-induced gut barrier dysfunction, dysbiosis, and endotoxemia prevent a return to full immune system health. The figure shows our current working hypothesis as to how B. burgdorferi infection changes a patient's health status, which is a change that may persist even after antibiotic treatment.
Immune Responses to Influenza Virus Infection and Immunization
The influenza infection mouse model serves as an outstanding example of how highly protective B cell responses are induced and maintained lifelong. In response to the infection, we see the activation of three waves of B cell responses:
- An initial activation of B-1 cells that secrete IgM as well as a neurotransmitter, acetylcholine.
- An early induction of so-called “extrafollicular B cell responses” that induce early protective IgM, IgG, and IgA antiviral antibody responses by plasmablasts, as well as the generation of early memory B cells.
- A robust CD4 T cell-dependent germinal center response in which B cells undergo clonal diversification to enrich the repertoire of B cells specific to the influenza virus, then differentiate into memory B cells and antibody-producing plasma cells (see figure below).
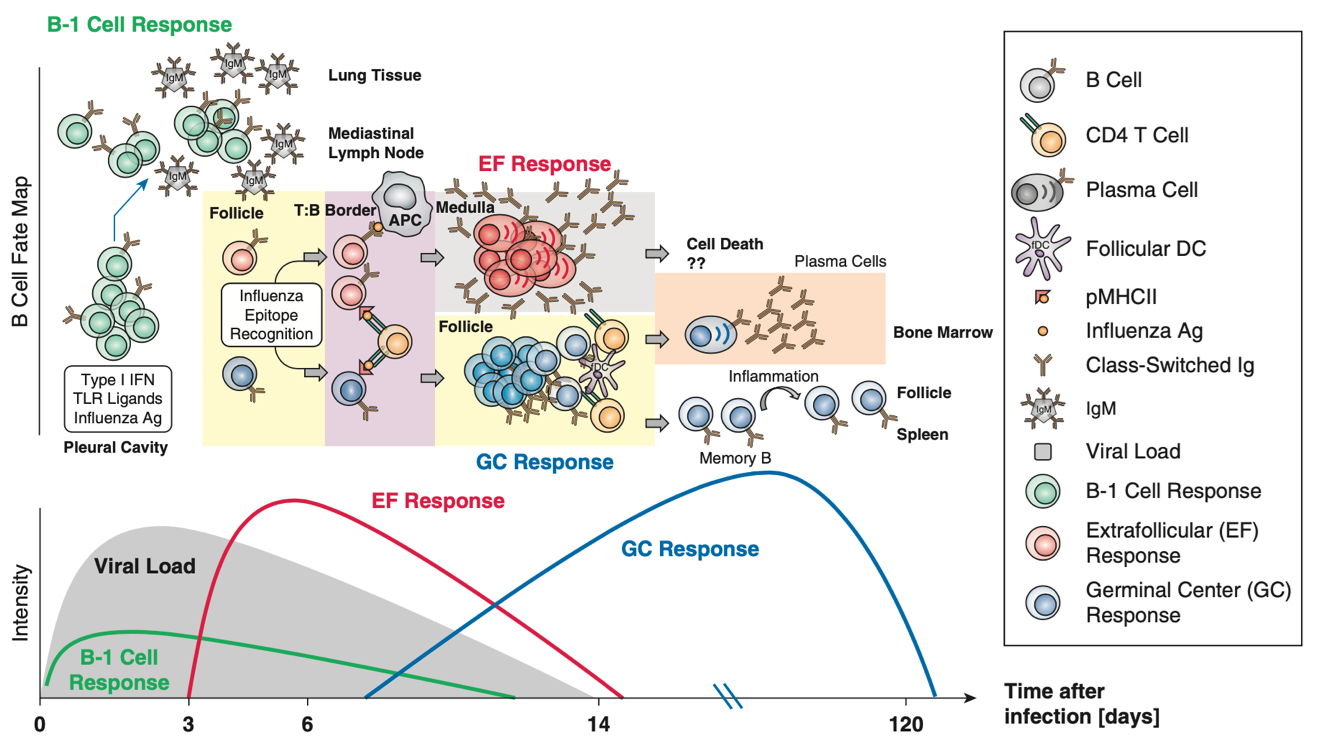
Figure from Lam JH and Baumgarth N, 2019. B cell responses to influenza occur in at least 3 waves: B-1, extrafollicular and germinal center responses, respectively, each contributing distinct protective response to the overall adaptive immune response.
A focus of our lab has been on the early B cell responses to influenza, both B-1 (see below) as well as extrafollicular responses. These responses are much less well understood than germinal center responses, yet our studies (Baumgarth, N et al. 2000, Choi, YS et al, 2008; Lam JH and Baumgarth N, 2023) have clearly shown that they are critical for protection and survival. Interestingly, our studies have also shown that extrafollicular B cell responses are not induced after subcutaneous immunization of the mice with virus particles in the adjuvant alum but induction can be rescued by providing inflammatory signals through the provision of toll-like receptor (TLR) agonists.
We aim to define how inflammatory signals can enhance extrafollicular B cell responses and what effect this may have on the subsequent germinal center response. This is important because the reasons why infections often provide better immunity than immunization are not fully understood. We also like to learn more about the memory responses that develop outside the germinal center and study their induction and maintenance. The results of our studies could provide some clues as to how extrafollicular responses help effective immune responses to develop and how we may need to alter vaccines to better mimic such responses.
Innate-like B Cell Responses and Natural Antibodies
B-1 Cell Responses to Influenza Virus
While T and B cells are components of the adaptive immune system, a small subset of B cells (termed B-1 cells) is considered an "innate-like" lymphocyte. This is because B-1 cells express aspects of the adaptive immune system: an antigen-receptor that relies on gene rearrangement and the innate immune system, rapid responses to inflammatory signals, and a BCR that can bind to pathogen-associated molecular patterns as well as altered self-antigens.
We first described how B-1 cells provide “natural” antibodies that can bind to the influenza virus, even in the complete absence of prior exposure to this pathogen that does not usually infect mice (Baumgarth N, 1999), providing a true “first line” of antibody-mediated defense that helps reduce initial virus burden until adaptive immune responses are initiated. These natural antibodies seem to bind to both “altered self” as well as foreign antigens and seem to be highly evolutionarily conserved (Baumgarth N, 2011).
We also showed that in addition to this passive mode of protection, B-1 cells also actively participate in the response to influenza infection by migrating to the draining lymph nodes, where they secrete both influenza-binding and non-binding IgM. Their responses are driven by innate-like signals, including type I interferon and TLR signals (Choi YS and Baumgarth N, 2008; Waffarn EE et al. 2015; Savage HP et al. 2019).
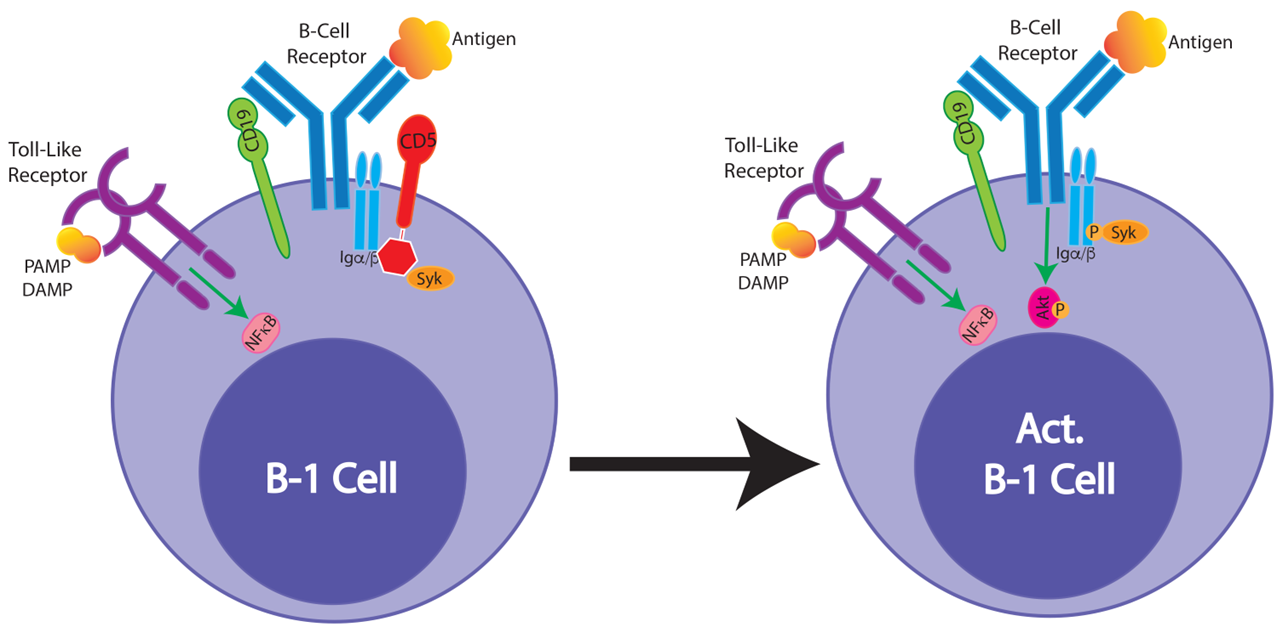
Figure from Smith FL and Baumgarth N, 2019. B-1 cells respond strongly to innate signals, such as TLR stimulation but various inhibitors are preventing BCR-mediated activation. Our studies have shown that TLR stimulation downregulates the inhibitor CD5 on B-1 cells, raising the possibility that the innate signals enable subsequent antigen-specific stimulation.
Unresolved questions we would like to answer are: how are B-1 cells regulated to provide active immune protection when they do not respond to B cell receptor stimulation with clonal expansion, but instead rely on innate signals for their activation? Are these truly innate responses? Is their specificity related to their ability to respond to an infection? A clue might be our recent findings: following TLR stimulation, the B cell receptor inhibitor CD5 is lost on B-1 cells.
B-1 Cells and Natural Antibody Production
Our early influenza infection studies support the critical function of natural antibodies in immune protection. Others have also shown that natural IgM prevents autoimmune response induction—a finding we have confirmed (Nguyen et al. 2015). We have worked to identify the cellular sources of natural IgM and found it to be generated by B-1-derived plasma cells in the spleen and bone marrow, but not in other tissues where B-1 cells reside (body cavities). We also found that there are two types of plasma cells, including one that does not express, nor require, Blimp-1 (Savage et al. 2017). And perhaps most surprisingly, we found that CD4 T cells are needed for natural IgM production by bone marrow B-1 plasma cells (Smith et al. 2023). Yet how CD4 T cells help these responses remains an unanswered question we would like to address.
There are many other unanswered questions that we would like to tackle. For example, what is the importance of generating IgM versus IgG? Why is it IgM antibodies are induced early in infection but typically not later? When IgM is induced later in an immune response to a pathogen, for example, during B. burgdorferi infection (Hastey et al. 2023), is that detrimental to the immune response? What is the role of the FcmR and how is its chaperone function that we identified (Nguyen et al. 2017) related to B cell response regulation?
B Cells and the Production of the Neurotransmitter and Immune Regulator Acetylcholine in Influenza Infection
Although B cells are best known for their ability to generate antibodies, they can also secrete other factors, including cytokines and metabolites, including acetylcholine: an important neurotransmitter that has been shown more recently to also have immune regulatory functions. Our recent studies have shown that B cells expressing the enzyme responsible for the generation of acetylcholine (choline acetyltransferase, or ChAT) are important for the early regulation of inflammation (Cembellin Prieto A et al, 2024, in revision). Specifically, we showed that B cell-derived acetylcholine inhibits macrophages from producing TNFa: a cytokine that controls influenza virus replication by inducing cell death of virus-infected cells and also acts to activate the immune system (“pro-inflammatory cytokine).
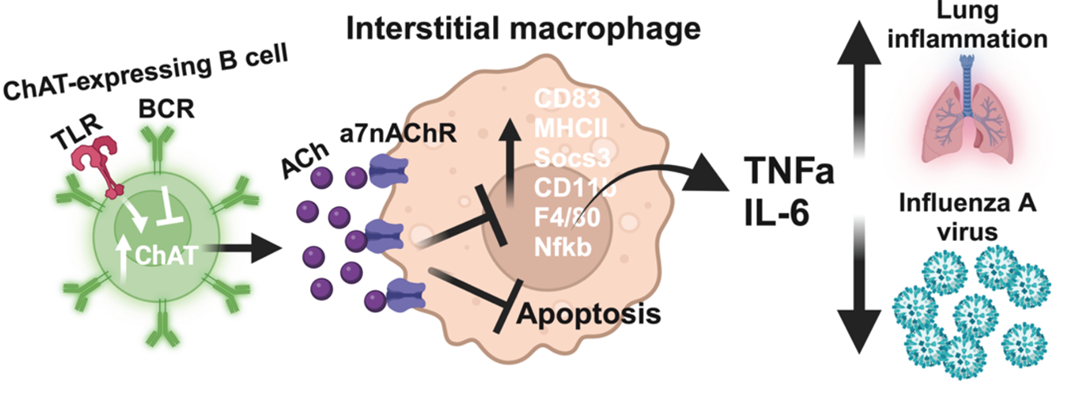
Model of how lung interstitial macrophages are controlled by B cells secreting acetylcholine early in response to influenza virus infection. Ach-producing B cells help balance the need to control early virus production with the need to control overshooting inflammation that could result in lung tissue damage (Figure from Cembellin Prieto A et al, 2024 in revision).
Given the importance of balancing the control of virus replication, while also avoiding lung tissue damage from the infection by an overshooting inflammatory response, identification of this new regulatory axis of lung immunity might be a novel target of therapies aimed at reducing early lung damage and inflammation after respiratory tract infections.
We are excited to learn more about what controls B cell production of acetylcholine, and when and how the function of B cells is activated. It is notable that many, but not all, B cells capable of generating acetylcholine are B-1 cells, adding a novel immediate-early immune function to this understudied B cell population.